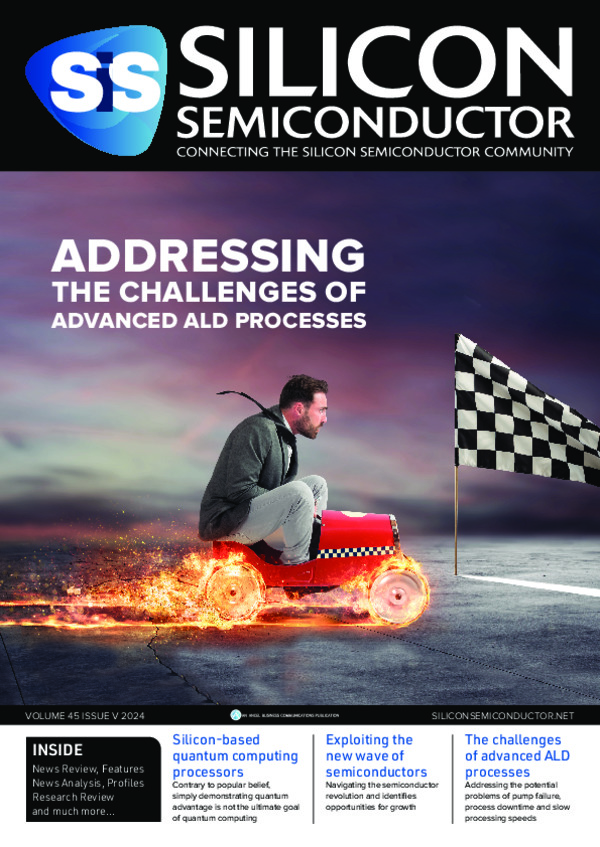
The crucial role of ADCs and DACs in scaling quantum computing
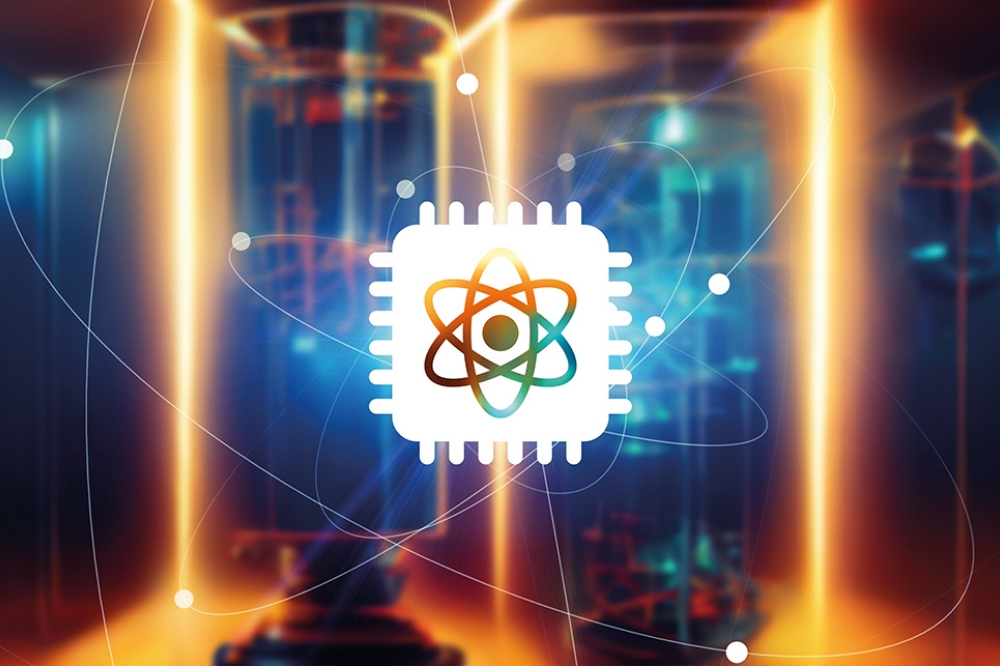
In recent years there has been a surge in interest surrounding the topic of quantum computing and heightened anticipation about its potential to revolutionise the technology world. However, its future relies heavily on the complex interplay with a broader set of factors. While qubits may steal the spotlight, the Analog-to-Digital Converters (ADCs) and Digital-to-Analog Converters (DACs) operating behind the scenes play a vital role.
By Chris Morrison, Director of Product Marketing, Agile AnalogThe crucial role of ADCs and DACs in scaling quantum computing
Firstly, let’s give a brief overview of what’s involved with quantum computing. At the heart of quantum computing lies the quantum elements such as qubits and gates.
Qubits are the fundamental units of quantum information. Unlike the classical bits we use in conventional computers, which can only exist in a binary state of 0 or 1 at a given moment, qubits can simultaneously occupy states of 0, 1, or even a combination of the two, thanks to a quantum phenomenon known as superposition.
This unique property of superposition allows qubits to perform parallel calculations, offering ground-breaking potential for quantum computing applications. Whereas classical bits can only represent one state at a time, qubits can exploit the richness of quantum mechanics to process a multitude of information simultaneously, offering exciting prospects for solving complex problems.
A quantum gate (or quantum logic gate) is a basic quantum circuit operating on a small number of qubits. Quantum logic gates are the building blocks of quantum circuits, like classical logic gates are for conventional digital circuits. They manipulate qubits to perform quantum operations.
Below is a short description of different types of quantum
computer.
Superconducting quantum computers use superconducting
circuits to create qubits. In order to preserve their quantum characteristics,
the circuits are cooled to incredibly low temperatures. Superconducting quantum
computers are comparatively advanced and capable of carrying out a large number
of computations. However, they are highly sensitive to their environment and
can be difficult to scale to larger sizes.
Trapped ion quantum computers use trapped ions to produce qubits. Ions are atoms that have lost or gained electrons, and they can be trapped in an electromagnetic field. Though complex to construct and operate, trapped ion quantum computers are nonetheless a relatively mature technology.
Photonic quantum computers use photons (particles of light) to form qubits. These are still in the early stages of development but are thought to be scalable because photons can be easily delivered over great distances with the use of optical fibres. In addition to being challenging to build, photonic quantum computers require specialized hardware.
Neutral atom quantum computers use neutral atoms (atoms that have not lost or gained electrons) to create qubits. These too are in the early phases of development and have the potential to be highly scalable. This is due to the ease with which lasers may be used to trap neutral atoms. Neutral atom quantum computers are also complex to build and need specialized hardware.
Why are ADCs and DACs needed?
The control and measurement of qubits often involve
converting between analog and digital signals. This is where ADCs and DACs come
into play.
Role of Analog-to-Digital Converters (ADCs)
ADCs are instrumental in quantum control, converting
analog signals from the measured qubits into digital data that can be processed
by standard control systems. Advances in ADC technology have led to the
development of high-resolution converters capable of accurately capturing
quantum signals with minimal noise and distortion. These ADCs also enable
precise measurement of quantum states, providing the feedback necessary for
real-time control and error correction in quantum systems.
ADCs for sensitive readout
After performing quantum computations, the qubit states
need to be read out. This involves measuring weak signals that are converted to
digital form using ADCs. Each qubit needs between 1 and 3 ADCs depending on the
implementation. Key considerations for ADCs in this context include:
£ High sensitivity and dynamic range
The ADCs must be highly sensitive to capture the faint
signals from the qubits and possess a wide dynamic range to accommodate the
full range of potential values. In general, these control signals need to be
between 5 and 12 bits of resolution.
£ High speed
Efficient readout relies on ADCs with fast sampling
rates to capture the rapid changes in the qubit states. With today’s
technology, quantum computers are moving from ADC sampling rates of 10s of MSPS
up to 100s of MSPS, or even 1GSPS.
Role of Digital-to-Analog Converters (DACs)
DACs play a key role in quantum control by converting
digital control signals into analog voltages or microwave pulses used to
manipulate qubits and implement quantum gates. Recent developments in DAC
technology have focused on increasing speed and precision, allowing for faster
and more accurate control of quantum operations. These DACs enable the
implementation of complex pulse sequences and control algorithms, leading to
improved gate reliability and quantum algorithm performance.
DACs for precise control
Quantum gates, the fundamental operations performed on
qubits, are implemented through carefully designed control signals. There
is typically a requirement for 2 to 5 DACs per qubit. DACs with the following features are essential:
£ High resolution and speed
Precise manipulation of qubit states requires DACs
with high resolution (8-12 bits) to accurately represent the complex
control signals and fast settling times (nanoseconds or faster) to
ensure minimal signal distortion. Again, with today’s technology, quantum
computers are moving from DAC sampling rates of 10s of MSPS up to 100s of MSPS,
or even 1GSPS.
£ Low noise
Any noise introduced by the DAC can lead to errors in the
control signals, compromising the fidelity of quantum operations.
Requirements of different qubit technologies
Whilst all forms of quantum computers make extensive use
of ADCs and DACs, each particular quantum computing topology has its own
specific requirements. The requirements for ADCs and DACs can vary. For example:
Superconducting qubits
£ ADCs
Low-noise, high-bandwidth ADCs are required to measure the
weak signals from the resonators used for readout.
£ DACs
High-fidelity voltage or current DACs are needed to
generate the precise microwave control signals.
Trapped ion qubits
£ ADCs
High-sensitivity, high-speed ADCs are needed to convert
the weak fluorescence signals from the trapped ions during readout.
£ DACs
High-speed, high-resolution DACs are used to modulate the
intensity and phase of the laser beams for control.
Challenges of ADC and DAC integration
Integrating high-performance ADCs and DACs into quantum
computing systems presents several challenges:
£ Miniaturization
As the number of qubits increases, the need
for compact and scalable ADCs and DACs becomes crucial. For example,
if you need 3 ADCs and 5 DACs for each qubit and you want 1,000 qubits, you
need 8,000 converters. So, 1 million qubits need 8 million converters!
£ Integration
Seamless integration of these converters with the qubit
control and readout electronics is essential for efficient system operation.
£ Power consumption
Maintaining low power consumption is absolutely critical
to the overall energy efficiency of quantum computers. Taking the example of
8,000 or
8 million converters, if these all consume 1mW then this leads to a total
consumption of 8 Watts or 8,000 Watts. This all generates significant heat and
modern cryostats are only capable of maintaining their temperature with between
2 and 5 Watts of internal dissipation.
Control electronics
Control electronics interface with quantum systems to
provide precise control and measurement capabilities.
£ Issues with external control electronics
Currently, most quantum computers have their control
circuitry, including ADCs and DACs, housed outside the ultra-cold cryogenic
chamber (cryostat) where the qubits reside. This approach, while functional for
small-scale systems, presents a significant bottleneck for scaling to thousands
or even millions of qubits.
£ Limited scalability
The number of qubits that can be controlled is restricted
by the physical limitations of the cryostat. Routing numerous control
cables for each qubit, including those for ADCs and DACs, becomes impractical
and cumbersome as the number of qubits increases.
£ Signal degradation
Long control cables introduce signal degradation and
noise, leading to errors in qubit control and readout. These errors become more
pronounced with increasing cable lengths, hindering the fidelity of quantum
operations.
£ Increased complexity
Managing and routing numerous control cables outside the
cryostat adds to the complexity of the system, making it difficult to
maintain and scale.
Advantages of integrating control electronics within
the cryostat
Integrating control electronics, especially ADCs and DACs, directly within the cryostat holds the key to overcoming the scalability bottleneck and paving the way for building
larger and more powerful quantum computers. There are several advantages:
£ Reduced signal degradation
By placing the ADCs and DACs closer to the qubits, signal losses and noise are minimized, leading to improved control and readout fidelity.
£ Enhanced scalability
With on-chip analog control electronics, the number of qubits that can be controlled is no longer limited by the number of cryostat feedthroughs. This allows for the construction of larger and more complex quantum circuits. Digital control circuity can be integrated within the cryostat or, due to the inherent noise immunity for digital signals, remain outside.
£ Simplified system design
Integrating the analog control electronics within the cryostat reduces the complexity of the system, making it easier to manage and maintain.
Challenges and considerations
While integrating ADCs and DACs within the cryostat offers
significant advantages, several challenges need to be addressed:
£ Harsh cryogenic environment
ADCs and DACs designed for room temperature operation need to be adapted to function reliably at cryogenic temperatures, normally around 4 Kelvin. This involves using specialized circuit design techniques.
Whilst semiconductor process technologies are typically characterized for operation from -40C to 125C, new models are required to design at these low temperatures. In addition, changes need to be made to the underlying transistors provided by the foundry to achieve optimal performance at these low temperatures.
£ Limited space
Integrating numerous ADCs and DACs within the limited
space available near the qubits requires miniaturization and high-density
packaging solutions.
£ Power consumption
Managing heat dissipation within the cryostat is
crucial. Low-power ADCs and DACs are essential to avoid compromising
the thermal stability of the system.
Scaling quantum computers
To achieve their true potential, there is a need to
dramatically increase the number of qubits, from the several hundred that is possible today to millions.
These qubits have to be controlled, and by generating semiconductor IP that can operate at cryogenic temperatures, quantum computing developers can quickly design their own control ASICs that can be co-located with the qubits in the cryostat.
In the world of quantum computing, where operational qubits demand temperatures even lower than 4K, co-locating the analog control electronics close to the qubits within the cryostat is key for quantum computer scaling.
Advances in ADCs and DACs
As the field of quantum computing continues to evolve, the
need for high-fidelity, low-noise, and high-speed analog components will only
become more critical. Agile Analog is exploring opportunities to develop a
range of cryogenic ADCs and DACs. The design team has acquired experience of
some of the challenges involved during a project with sureCore, the ultra-low
power embedded memory specialist, that delivered a cryogenic control ASIC in
Global Foundries 22FDX technology, as part of an Innovate UK funded project.
Conclusion
The path towards a robust and scalable quantum computer is
paved not only by advancements in qubit technology but also by the development
of high-performance and reliable analog components. As quantum computers grow
in size and complexity, the demands on the analog components increase. From
generating control signals to amplifying weak qubit states, these components
play a central role at various stages of the quantum computing stack. ADCs and
DACs are poised to play a vital part in unlocking the full potential of quantum
computing. The continuous development of high-performance analog electronics in
tandem with advances in qubit technology will drive the evolution of quantum
computing towards increasingly complex and powerful applications.