
New graphene hall effect sensors deliver high performance
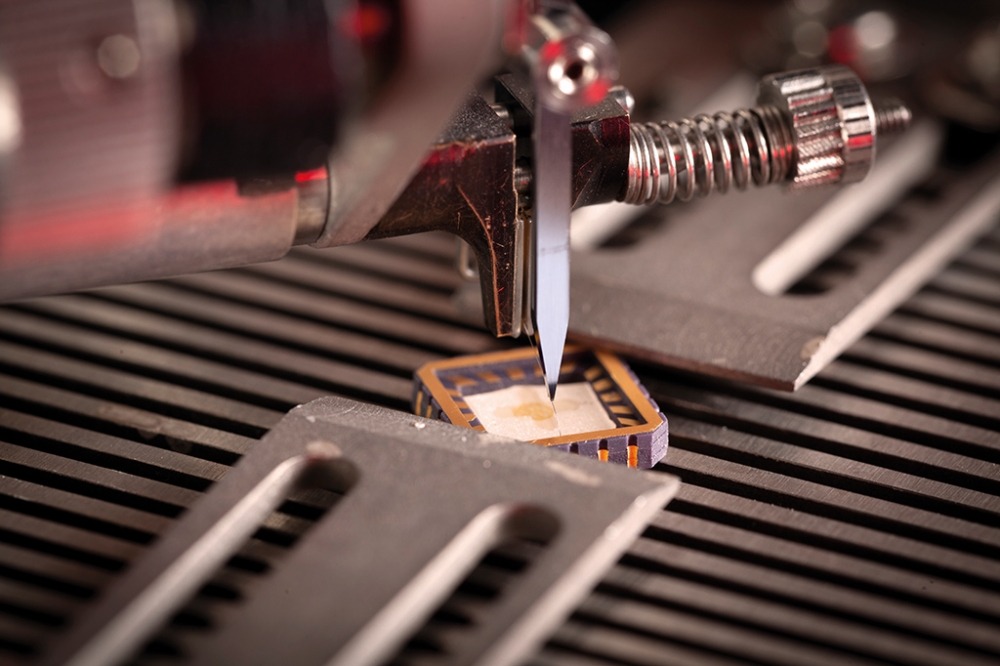
Graphene has long tantalized electronic device developers thanks to its unique 2D capabilities. But harvesting graphene while maintaining its most sought-after properties has proved elusive, delaying adoption. UK-based Paragraf and CEO Dr. Simon Thomas say the company is poised to disrupt the status quo with unique products including robust Hall Effect sensors; key to its success is Paragraf’s new method of manufacturing graphene at scale to support commercialisation in electronic applications.
THERE HAS, for a long time now, been a buzz around graphene, the one-atom-thick allotrope of carbon that boasts some extraordinary properties around thinness, flexibility, robustness and electrical conductivity. The hyperbole has seen graphene grandiosely described as a ‘wonder material’ that is going to find transformative applications across the electronics sector.
However, living up to that heightened sense of expectation has not proved easy. To date, graphene as a material has largely failed to deliver on its potential in electronic device applications, primarily because of how it is manufactured and processed. With that in mind, a pertinent question needs to be asked: will graphene ever deliver on the hype?
To find an answer to that, we need to take a step back and look at some of the challenges of manufacturing graphene for use in the electronics sector. Most commercially produced graphene is either created by exfoliating graphite or forming the graphene onto a metal substrate – most commonly a copper foil. While exfoliating graphite produces good quality graphene, the graphene produced is typically very small in area, multi-layered, non-homogeneous and non-customizable; therefore, it is not suitable for electronic devices other than at the R&D level.
Electrical measurements of a Hall Effect sensor on wafer
Graphene grown on copper foil, meanwhile, needs to be transferred onto an electronics compatible substrate after being synthesised, involving various wet and dry transfer processes that can affect how the graphene functions in an electronic device. It is also contaminated by the copper. These challenges mean there has been a lack of contamination-free, transfer-free, large-area graphene available in the market, and adoption in mass-market electronics remains slow. New solutions are clearly required if graphene is to make its mark in the electronics sector.
Novel technique holds real promise
Step forward Paragraf, who have caused quite a stir by developing an innovative method of producing graphene at scale. The excitement relates to a scalable and patented technology that uses processes to allow the manufacturing of large-area, high-quality graphene (currently up to 8” diameter).
The approach uses a modified deposition method that removes the need for the transfer processes commonly applied in most large area graphene synthesis methods. Therefore, the graphene can be produced in a uniform, single layer directly on a wide range of substrates, including silicon, silicon carbide, sapphire, gallium nitride, and other semiconductor-compatible substrates. It is also free from metallic contamination.
On the face of it, then, this scalable approach overcomes many of the challenges for developing graphene for electronics applications. The next step is to make the most of these advances by identifying suitable applications and bringing commercially viable products to market.
This process has already begun, with Paragraf’s scientists and engineers developing a new graphene-based Hall Effect sensor. Existing Hall Effect devices, made from silicon, are primarily used for magnetic sensing. However, they have limitations in terms of sensitivity, accuracy and magnetic field range. Paragraf believes its graphene device will overcome many of these performance inhibiting parameters.
So, let’s look at those claims in a bit more detail, starting with the specifics of why graphene, as a material, is so suitable for use in a Hall Effect sensor. Crucially, graphene has a property called sheet carrier concentration: essentially the number of electrons available to move through the material carrying charge, which is very low. When configuring the material to interact with other electrical or magnetic fields, such as in a Hall Effect sensor, this property directly translates to a very high level of sensitivity in the device. In real terms this can be 30 to 50 times more sensitive than a standard semiconductor such as silicon.
Also, importantly, graphene is a two-dimensional material, meaning it does not exhibit the same directional properties as thicker, or bulk materials. For Hall Effect sensors this is particularly useful: one of the issues with traditional devices is the thickness of the sensing material, which causes the sensing layer to be three-dimensional. This causes field components that are not perpendicular to the sensing direction to also be sensed, and as a result, false signals are produced. This is known as the planar Hall effect. The lack of a planar Hall Effect in Paragraf sensors is due to the inherent thinness of monolayer graphene, so these errors are removed, allowing for higher precision mapping of magnetic fields.
Paragraf scientists performing electrical measurements of packaged devices
Also, graphene is very robust and does not suffer from the thermal impacts common with conventional semiconductor devices. This allows the sensor to work at more extreme temperatures, much higher and much lower than standard silicon semiconductor devices. They can be used in extreme cryogenic temperatures at conditions of less than -271° C (1.8 K) and currently up to 80° C (353 K), meaning they can be used in superconducting environments while remaining highly sensitive.
As well as being resistant to thermal shock, no electrostatic discharge protection is required, so the sensors can be plugged straight into the mains (220V) without any adverse effects occurring. This makes the sensors easier to handle in industrial environments.
The graphene-based Hall Effect sensors also have very low power dissipation – of the order of picowatts (pW) with nanoamperes (nA) drive current – so they will not heat cryogenic environments and will save energy compared to other Hall Effect sensors.
These superior performance properties mean the Paragraf sensor provides the answer in a broad range of applications and technologies, without the restrictions of existing devices, says Dr Simon Thomas, Paragraf CEO.
“The resolution, combined with the lack of planar Hall Effect, gives it a very high accuracy capability, way beyond what is possible with any other Hall sensor on the market. Then, considering this comes in a small package which is robust in extreme conditions, you end up with a very attractive device which offers comparable performance levels to fluxgate sensors and NMR probes,” he explained.
Field testing provides validation
The technical characteristics of the Paragraf sensor have been proven during field tests with several partners. For instance, Paragraf is working with the Magnetic Measurement Laboratory of the European Organization for Nuclear Research (CERN), which has tested sensors to see if they could accurately map the magnetic fields in electromagnets used within CERN’s unique set up.
The field tests show definitively that Paragraf’s sensor has a negligible planar Hall Effect and therefore only measures field components along the desired axis of measurement. CERN is looking to build a stacked mapping system of Hall Effect sensors that can provide very accurate magnetic field measurements using Paragraf’s devices.
Analysis of devices on wafer utilizing an atomic force microscope
Paragraf has also been working with the UK’s National Physical Laboratory to investigate the suitability of sensors in extremely harsh environments and with Queen Mary University of London to study the potential of graphene to replace Indium Tin Oxide in electronic devices.
“The Hall Effect sensor has demonstrated its versatility in a range of magnetic field measurement applications, and this is set to continue in the coming years. The operating temperature range and high sensitivity of the sensor open up application areas not previously possible,” added Thomas.
“One example is the aerospace industry where they could be embedded into aircraft engines for the continuous monitoring of faults in real-time.
Also, once the testing work with the NPL is complete, and the sensor is shown to be capable of operating in harsh radiation environments, applications could include use as positional sensors within nuclear-decommissioning robots and space satellites,” he concluded.
Paragraf scientists performing experiments on devices inside a glovebox